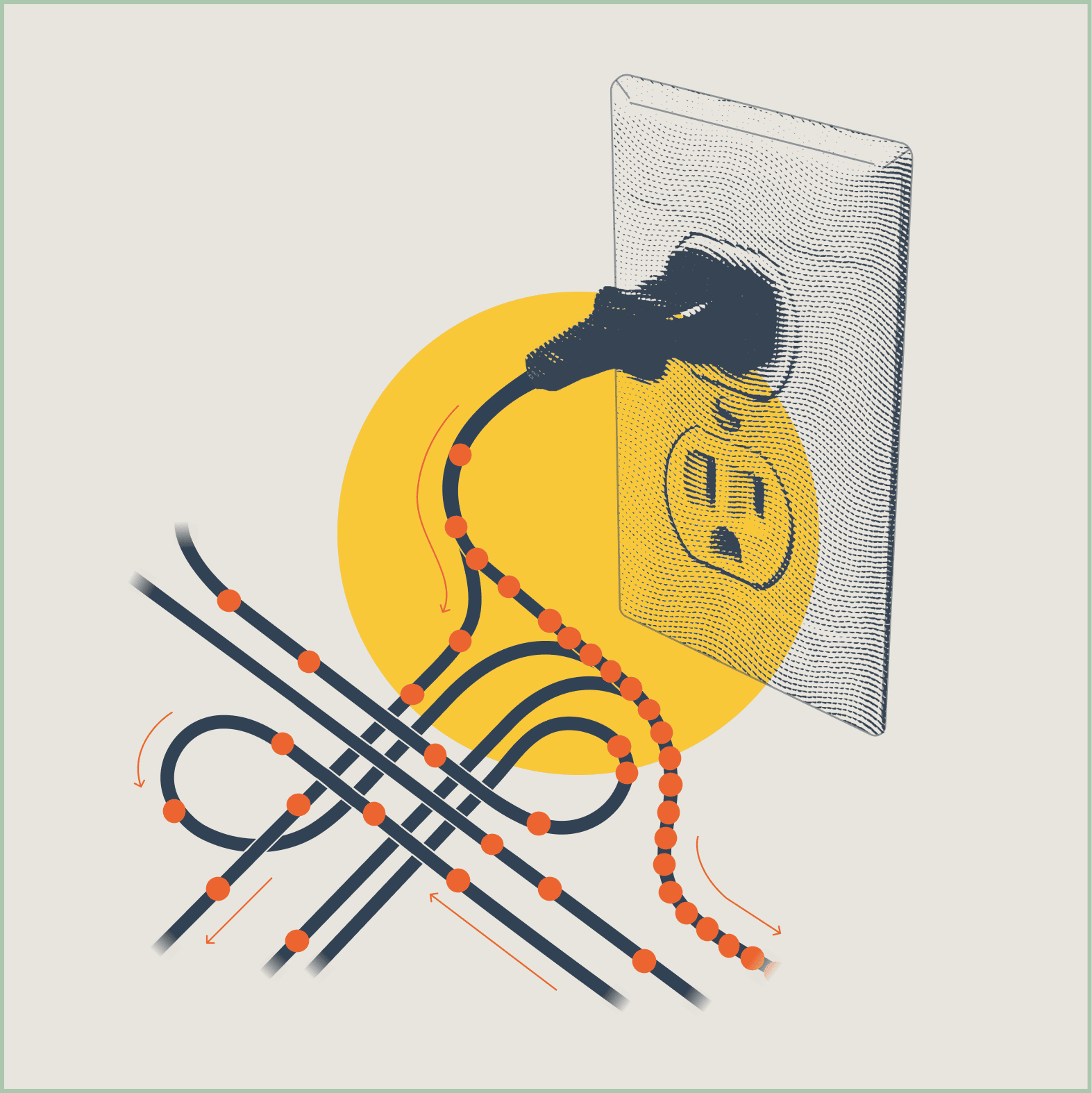
Introduction: Understanding Generation Interconnection
For anyone interested in the shift towards clean energy, the term “Generation Interconnection” is likely familiar. It is a process through which transmission network operators ensure that adding new generation sources doesn’t compromise the power grid’s reliability and stability. This process involves several stages with intricate and often computationally intensive studies to assess the potential impact of proposed generation interconnection projects. Typically, projects are placed in a queue based on their submission time for connecting to the transmission system. The specific procedures vary by geographical location, as each region’s system operator governs the processes. For instance, if you plan to build a solar power plant in Virginia, you must navigate PJM’s generation interconnection process, while in Arkansas, you must adhere to MISO’s regulations.
The State of Queue Backlogs
It’s no secret that these queues are steadily growing, and the pace at which projects are leaving the queue isn’t keeping up with new entries. According to a recent report from the Lawrence Berkeley National Laboratory, the total capacity of energy projects in U.S. interconnection queues surged by 40% year-over-year in 2022. These queues now hold over 1,350 GW of generation capacity and 680 GW of storage projects awaiting approval for connection. There are ongoing discussions about the underlying causes of this issue and potential remedies for the backlog. While there are many speculations and uncertainties about whether proposed queue structure reforms will alleviate the problem, it’s almost certain that the interconnection process and the associated studies will become more sophisticated as the backlog continues to swell.
Key Stakeholders and Their Objectives
Every stakeholder involved in the generation interconnection process has distinct objectives, but they all rely on the same set of interconnection reliability studies. For instance, transmission operators and owners aim to safeguard grid reliability while project developers seek to optimize their return on investment and identify the best project locations. Determining the “best” location depends on various factors, primarily driven by overall project cost and feasibility. Among these factors, interconnection costs play a pivotal role. Beyond the evident construction expenses of a solar power plant, there’s the critical “affected network upgrade cost” linked to each project. This cost can be the make-or-break factor for a project’s viability. While delving into the intricate details of what constitutes network upgrade costs could fill numerous posts and articles, at its core, it represents each developer’s equitable contribution to upgrading the transmission network, ensuring it can accommodate new generation without compromising reliability.
Demystifying Injection Studies
The power grid is a complex system made up of numerous components. Any change, whether it involves adding a new load or connecting a new generation source, requires a careful evaluation to assess its impacts on the system. In the industry, the collection of simulations and calculations used to determine the feasibility of connecting a specific generator to the network is commonly known as “injection studies.”
Now, let’s dive into injection studies, their significance, and what they entail. An injection study involves a series of power system simulations conducted under various stress conditions or contingencies. Its primary goal is to assess whether injecting power at a specific location within the network would negatively affect grid reliability and stability.
To grasp this concept better, we must first define “reliability” in this context. In an electric transmission system, each current-carrying equipment has a maximum threshold for the total current it can safely handle without overheating due to thermal losses. This threshold is often referred to as the equipment’s thermal capacity or rating and varies based on factors like material characteristics and nominal voltage. Strict standards and regulations govern how much current a given piece of equipment can carry under normal operating conditions to ensure safe and reliable system performance.
Think of the transmission network as a network of highways with existing traffic or load.
When you introduce new energy sources capable of injecting additional current into the network, you bring it closer to the maximum capacity for some already heavily loaded equipment. The injection study’s purpose is twofold: first, to determine the existing transmission network’s available capacity before overloading any equipment, and second, to identify which equipment would become overloaded and require upgrades if a specific amount of power was to be injected at a designated point of interconnection (POI).
Understanding the Constraints
It’s important to note that this discussion focuses solely on thermal constraints within the transmission network. There are additional constraints, such as voltage, frequency stability, and short circuit which necessitate their own set of studies to assess the impact of new generation sources.
The Nuances of Network Upgrade Costs
Now that we’ve covered injection studies, let’s delve into why things are getting more complex as additional projects enter the GI queue. As previously mentioned, when simulation results indicate that one or more transmission equipment could be overloaded due to the additional current injected by a proposed generation project, upgrades are necessary to ensure safe and reliable grid connection. These upgrades can range from hundreds of thousands to millions of dollars, depending on the equipment involved.
High-voltage assets like 500kV transformers and transmission lines come with hefty price tags for upgrades, whereas lower-voltage equipment typically incurs lower costs.
While guidelines do exist for estimating the network upgrade costs that may be incurred, the primary responsibility for calculating the overall network upgrade cost rests with the transmission owner. Subsequently, this calculated cost is reported to the transmission operator, who oversees the GI queue. Determining the total cost of the upgrade itself is not inherently challenging; rather, the complexity lies in the equitable distribution of this cost among interconnection customers. This complexity arises because a single piece of equipment often undergoes multiple impacts from various GI projects, as multiple developers seek interconnection within a specific geographic area. Fortunately, it’s technically feasible to precisely calculate each project’s contribution to the equipment overload and allocate the upgrade costs accordingly.
Impact of Project Withdrawals
Some developers will decide to withdraw their projects from the queue if assigned a high network upgrade cost, as they aim to minimize expenses. This withdrawal has an immediate impact on cost allocation for other projects, as the canceled project’s share must be redistributed among the remaining ones. What complicates matters further is that developers typically don’t receive immediate notifications of other developers’ decisions to continue or withdraw. These determinations are usually made at predefined phases or decision points set by the queue manager (in this case, the transmission operator).
Consequently, successfully siting a GI project becomes akin to a chess game, requiring a degree of predicting competitors’ likely moves.
The Steps to Siting a GI Project
Let’s dive into the process of finding the optimal location within the transmission network to interconnect your generation project. To make it more concrete, let’s imagine we’re a utility-scale solar development company aiming to build a solar farm that connects to the existing transmission network. For this scenario, we’ve chosen to operate within the MISO footprint, which means we’ll be navigating the MISO cluster-based generation interconnection process.
Our first step is to determine if we can conduct the same studies that MISO will perform to assess the impact of our proposed project on the transmission network and estimate the associated network upgrade cost.
To begin, we need to evaluate two key aspects:
- Available transmission network capacity.
- Previously proposed GI projects.
The Importance of Modeling in GI Studies
Building a solid understanding of injection studies and transmission reliability studies requires extensive discussion, but at a high level, power system modeling is fundamental. Essentially, there’s an equivalent computer model for every piece of power system equipment found in real-world physical systems. For instance, transmission lines, transformers, and generators are represented by models that depict their unique characteristics within the power system.
Now, without diving too deeply into the complexities of transmission modeling for generation interconnection projects, it’s important to note that each project must be individually modeled in our studies. This raises several questions: Are we modeling all previously proposed GI projects, or just a subset? If it’s a subset, how do we select them? To what level of detail should we model them? What if the landscape of previously proposed projects changes (with some being withdrawn), and how do we update our models and assumptions?
Fortunately, some of these questions are addressed by the transmission operators like MISO, which provide a baseline model already encompassing all projects that entered the queue as part of a specific cluster. However, a common issue is that these models often assume all proposed projects will be built and connected to the grid simultaneously, which tends to overestimate the impact of new generation on transmission network capacity.
This leads generation developers to make adjustments to these models to create a more realistic version of the hypothetical grid, incorporating only a subset of proposed GI projects. So, the crucial question becomes: How do we select a subset of projects that is reasonable and, at the same time, conservative—ensuring that we don’t overestimate existing capacity by excluding a potential project that might have already claimed the transmission network capacity by the time our project seeks connection.
Conclusion: Navigating the GI Landscape
In the pursuit of a cleaner and more sustainable energy future, Generation Interconnection (GI) stands as a critical gateway. As we’ve journeyed through the intricacies of this process, we’ve uncovered the growing complexity of interconnection queues, the vital role of injection studies, the impact of project withdrawals, and the challenges associated with calculating network upgrade costs.
In this conclusion, we distill key takeaways to empower stakeholders navigating this evolving landscape:
- The Interconnection Challenge is Real and Growing: The energy transition has spurred a surge in GI queue entries, straining existing processes. It’s essential to anticipate ongoing complexities and work toward solutions that promote efficiency and reliability.
- Injection Studies are the Linchpin: Injection studies, assessing the impact of new generation on grid reliability, are pivotal. Understanding these studies and their results is crucial for developers, utilities, and grid operators.
- Collaboration is Key: As the GI landscape evolves, collaboration among stakeholders—developers, utilities, regulators, and technology providers—becomes paramount. Together, we can develop more robust and efficient GI processes.
A Glimpse into the Future
In this dynamic realm, adaptation and innovation are essential. The challenges are substantial, but so are the opportunities. By embracing the complexities of Generation Interconnection and exploring innovative solutions, we can accelerate the transition to a sustainable, clean energy future for all. Stay tuned as we continue our deep dive into the world of Generation Interconnection. Part 2 of this series will explore some of the existing tools and solutions to the challenges highlighted in this segment. Check out Part 2!
Loved the article? Hated it? Didn’t even read it?
We’d love to hear from you.